SECURING LNG’S LEADING ROLE ON THE GLOBAL ENERGY STAGE
Michael Pospisil P.E., Senior Engineer, and Rich Insull, P.E., Project Manager, Matrix PDM Engineering, detail the significance of life cycle analysis to helping secure LNG’s role in the future energy mix.
This article appears in LNG Industry’s April 2024 issue.
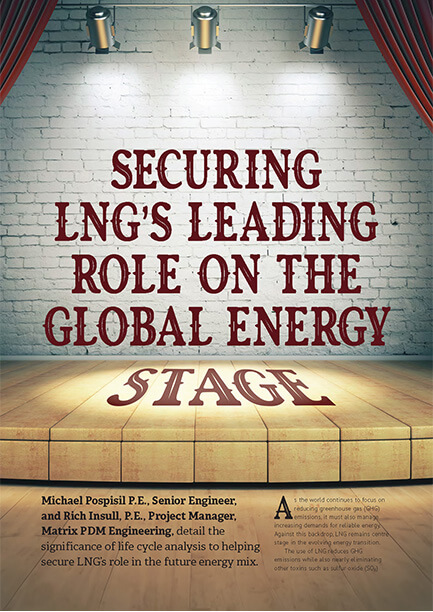
As the world continues to focus on reducing Greenhouse Gas (GHG) emissions, it must also manage increasing demands for reliable energy. Against this backdrop, Liquified Natural Gas (LNG) remains center stage in the evolving energy transition.
The use of LNG reduces GHG emissions while also nearly eliminating other toxins such as sulfur oxide (SOx) and nitrogen oxide (NOx). As such, it is the fuel of choice for diesel replacement in heavy horsepower applications. LNG is also a critical lower carbon solution that helps ensure reliable power for electricity, heating, and cooling in remote areas or during periods of peak demand such as extreme weather events.
Given its leading role, the inspection, maintenance, and repair of LNG tanks and terminals is vital to providing energy assurance through safe, ongoing operation. Ideally this work, referred to as Life Cycle Analysis (LCA), is completed as part of a planned Life Cycle Infrastructure Asset Management program for a facility, but it can sometimes be triggered because of unplanned events.
A real-world example
In January 2020, a series of earthquakes rocked the island of Puerto Rico, with the most significant being an M6.4 event that originated within 13 km of the EcoEléctrica LNG import terminal and power plant. A critical energy resource, the facility supplies natural gas fuel to produce up to 40 percent of the island’s total power, and its Natural Gas Combined Cycle power plant is the cleanest, most reliable source of energy for the island.
Seismic monitoring instrumentation on the EcoEléctrica tank indicated ground shaking caused by the M6.4 event produced accelerations on the structure that exceeded the seismic hazard developed for the original design.
As a result, EcoEléctrica was tasked with responding to multiple inquiries from United States (U.S.) regulatory bodies including the Federal Energy Regulatory Commission (FERC), Department of Transportation Pipeline and Hazardous Materials Safety Administration (PHMSA), U.S. Coast Guard (USCG), and U.S. Geological Survey (USGS). In question were the short-term and long-term risks associated with the facility’s 160,000 cu. meter double-containment LNG storage tank.
For answers, EcoEléctrica turned to Matrix PDM Engineering (Matrix), whose engineers possess extensive expertise in cryogenic tank design, and whose predecessor firm had designed, fabricated, and erected the EcoEléctrica tank which was commissioned in the early 2000s.
Matrix conceptualized the problem and developed the methodology for the tank analysis considering input from engineering seismologists, EcoEléctrica, and technical staff at regulatory agencies. The Matrix engineering team conducted multiple seismic and structural analyses on the tank system. The first was a forensic analysis following the M6.4 event. This analysis consisted of a desktop study for the seismic and structural analysis of the tank for accelerations developed from the ground motion recorded during the M6.4 event. The team was able to verify that the tank system remained undamaged during the M6.4 event and was safe for continued operation. Sample results for modal response spectrum analysis of the fluid structure system can be seen in Figures 1 and 2.
Additionally, regulatory agencies implemented restrictions on the liquid level inventory until EcoEléctrica could satisfy concerns for plant and public safety due to potential increases in short and long-term seismic hazards because of the M6.4 event. The Matrix team performed multiple seismic and structural analyses for seismic hazards including those developed based on current standards, as well as potential hazards developed to consider elevated return periods. Several of the evaluations considered seismic hazards beyond what is currently required by U.S. LNG codes including 49 CFR Part 193.
Ultimately, the LNG tank and its prestressed concrete secondary container were deemed to have performed safely during the M6.4 Event. The Matrix analysis was also used to support regulatory approval for liquid level that the facility could use for continued operation.
Planned LCA
While an unplanned event resulted in the LCA performed at EcoEléctrica, given LNG’s role in achieving global energy goals, planned LCAs should be a priority with today’s owner/operators – especially with aging facilities – to ensure their facility’s safe, continued operation and compliance with changing regulations. In the U.S. alone, there are 107 active LNG facilities (excluding mobile, temporary, and satellite facilities), 70 of which were constructed between 1965 and 1995, according to PHMSA.
The remainder of this article presents a structured approach for performing an LCA on the cryogenic storage systems at these facilities, whether double-wall, single, or full containment.
While assessment methodologies are similar for different tank systems, each tank has its own characteristics and requires a facility-specific process. The complexity requires that all phases of an LCA be carefully planned and executed.
Phase 1: Data Collection
Assessment begins with collection of information such as:
- Past tank loading and unloading cycles.
- Design calculations
- Design and fabrication drawings.
- Construction documentation (including material certificates, material test reports, and weld procedures).
- Geotechnical reports, commissioning, operation, maintenance, repair, and modification records.
Certain facility operating information is also necessary, such as transport logs, which indicate loading/unloading information, temperature data, foundation settlement information, and historical tank vapor pressure information.
The operating history and anticipated past and future loading cycles form the basis for a Fit For Service (FFS) and Remaining Service Life (RSL) assessment.
Assumptions based on historical data of similar facilities and experience may be made if there are gaps in facility data or operating information.
Understanding the owner’s objectives is critical to this phase, as this will significantly influence the work required in phases 2 and 3. A longer life expectancy, for example, will require closer evaluation and potentially more repairs. Similarly, if Phase 2 analysis results in a long future life, the work in Phase 3 can be minimized.
Phase 2: Desktop Study
The desktop study involves data review and integration; tank stress and fatigue analyses; identification of critical areas to inspect and validate assumptions or fundings; development of procedures to enable tank entry; inspection; repairs and closure. Phase 2 also includes planning and scheduling activities.
Typical assessments are iterative, with various methods applied. The assessment is focused on highly stressed, fatigue-sensitive components of the inner tank, and covers aspects such as the foundation, insulation, penetrations, and platforms.
Critical components of the inner tank include the circumferential weld of the shell to the annular plate; shell penetrations; and circumferential lap weld of the bottom plate to the annular plate. Underpinning the assessment are:
- Finite Element Analyses (FEA) to identify regions that are susceptible to fatigue.
- Fatigue analyses using crack growth models and damage accumulation mechanisms to estimate consumed and remaining design life.
The spectrum of topics covered is vast, and a complete depiction of the entire analyses is impractical. Therefore, only a few items are presented here.
A key issue in FEA modeling is the need to correctly mimic the as-built conditions to accurately depict peak stresses.
Another issue is geometric Stress Concentration Factors (SCFs). Occasionally, the FEA model and mesh size is not adequate to model the peak stresses. In such cases, SCFs can be utilized to properly depict the values. The SCFs are based on published literature and experience of the analyst.
Fatigue is the driving mechanism in an LCA evaluation, as tanks are continually loaded and unloaded. Due to repeated or fluctuating stresses, pre-existing minute cracks in material grow. A fatigue sensitive component will fail when these cracks propagate to a level that cannot be sustained.
Two approaches can be used for fatigue. The first is an S-N Curve Approach, where there is a finite number of cycles at a stress range at which a component fails. These curves exist for both smooth bar specimens and welded joints. For a specific joint under consideration:
- Stress ranges are determined using loads in a load cycle.
- The number of cycles at a joint for each load/unload cycle are identified using the loading regime.
- For every stress range associated with a load cycle, the number of cycles to failure is determined from published S-N Curves.
The ratio of cycles in the second and third items yields a damage fraction for a load cycle. Using a cumulative damage rule, such as the Miner’s rule, one can determine the life of the joint under consideration. The cumulative damage fraction has two parts: life consumed and life remaining. Once all components have been evaluated, the minimum life remaining will yield the RSL.
Like an SCF, the surface profile of the welds used in construction amplifies the impact of fatigue. The impact is handled by using Fatigue Strength Reduction Factors (FSRF), which are selected by the analyst based on experience and as published in codes and standards.
In general, the S-N Curve approach yields an idea of the RSL of the components that make up the cryogenic storage tank. However, it does not provide any information on intermediate stages of propagation of a crack, due to the loading/unloading cycles.
A second approach is a Fracture Mechanics (FM) evaluation. The premise for an FM evaluation is the growth of a postulated flaw at a location under cyclic loading. For analyses, a flaw at a specific location can be characterized based on the detectability in the Non-Destructive Examination (NDE) method used, or conservatively based on a design standard. Considering a postulated crack and using an acceptable material crack growth model, such as the modified Paris Law, one can simulate crack growth with every load cycle. The process can be summarized using a Failure Assessment Diagram (FAD). Failure is considered when the crack becomes unstable per the FAD. This method can be used to gain additional insight into RSL.
Phase 3: Entry, Inspection, Reassessment, and Repairs
Once the assessment has been completed, inspection requirements and potential upgrades are defined with emphasis on components with limiting fatigue value. Inspection requirements are developed using experience, industry norms, and API inspection standards. The main consideration for upgrades is the increase in design life.
During Phase 3, emphasis is on identifying areas of concern; accessing and inspecting those areas; performing upgrades as needed; and returning the tank system to facility operations. Inspection data is typically used to validate the engineering analyses assumptions or may require them to be modified. Information from final analyses coupled with inspection data, facilitates the development of repairs and upgrades to meet future facility requirements.
The first step in Phase 3 consists of purging and safe isolation of the tank before entry. Subsequent tasks include removal of perlite from the suspended deck and interstitial areas; cutting of the door sheet area to facilitate movement of personnel and equipment; removal of the balance of perlite if required; and inspection of critical components. Repairs performed may include additional welds to the annular to the bottom plate weld; and removal and replacing of pumps, pump columns and pump column braces. Before the tank returns to service, insulation removed for repair and entry purposes is replaced, and the tank and associated piping is purged with nitrogen and then cooled down. All work performed during this phase must be performed using specifications, procedures, and drawings developed during the previous phase.
For owners of LNG facilities – especially those that have been in operation for 20 years or more – performing an LCA is imperative to ensure safe, ongoing operation and to securing LNG’s place on the global energy stage.
Note
The LCA should be performed by specialists that have practical experience in designing, constructing, and commissioning facilities; possess a background in NDE methods and techniques; have performed internal and external inspections; and are skilled in FFS and RLS assessment. Input from the operator’s engineering and operations personnel is also critical. The assessment should be performed system-by-system within the facility and include both desktop analyses and on-site inspection to achieve a successful outcome.
Reprinted with permission from LNG Industry. View this article as a PDF here.